Anyone who has installed a photovoltaic system in their own home may even use an energy storage system. In this example, it is an off-grid system equipped with two modules from the manufacturer Pylontech. The Pylontech US3000C batteries have an output voltage of 48V. The nominal capacity is 3500Wh. The installed cells are LiFePO4 cells and the usable capacity is specified as 3374Wh according to the data sheet. The batteries are designed to be connected in parallel with other Pylontech batteries. The internally installed BMS (battery management system) communicates with the other Pylontech battery modules via a so-called “link” interface. A battery configured as a “master” handles the data exchange with the inverter. Here, Pylontech provides the CAN or RS485 bus as an interface. However, if you want information about the individual cells (voltages, currents, charges, temperatures, etc.), there is another interface on each module called “Console”. This is an RS232 interface via which you can communicate directly with the battery’s BMS. This port is also used to update the firmware of the BMS. However, I STRONGLY advise against playing around with firmware updates and flash software. This is reserved for the manufacturer or the liable party.
However, as this interface also provides a lot of information about the cells installed in the battery, this is an interesting approach. Initially, I had a laptop connected to a terminal and was able to discover and monitor the individual cell voltages and, above all, the possibly different charge status of the modules connected in parallel. So I thought it would be a good idea to have this information available in my home automation system, where it could be visualized and used for control purposes.
As we geeks and technology enthusiasts are quite familiar with terms such as Homeassistant, Docker, Proxmox, HomeMatic, NodeRed etc., I thought that this data should also become entities in the Homeassistant. So a small new project was quickly created. My plan was to read the data from the serial interface and send it to the Home Assistant via MQTT.
But before I start disassembling the data strings that come out via the serial port, I’ll have a look at the search engines. Perhaps someone else has already dealt with this topic. And that’s exactly what happened. I found what I was looking for on GitHub under the term “pylontec2mqtt”. A project is hosted at https://github.com/irekzielinski/Pylontech-Battery-Monitoring that uses ESP8266 to collect the serial data from the port and sends it to the Homeassistant server via MQTT and Wifi. A fork with a further development of this project can be found at https://github.com/hidaba/PylontechMonitoring.
Why am I publishing the project here on the blog despite the simple replica? I have optimized the circuit a little and packed it into a layout and adapted the code a little. I would like to share the result here. It was important to me to have a sensible structure on a circuit board that is connected with a USB A-B cable for the power supply and a LAN-RJ45 cable for the data connection. I wanted to use a “solid” USB connector (not the fragile mini or micro USB connectors)
On a breadboard and with the usual development boards, I quickly “knitted together” a functional model so that I could adapt the software to it.

So I first created a circuit diagram from the sketches in the Git project. There is a “real” RS232 level at the “Console” interface, which is converted to a 5V TTL via the MAX3232 IC. The BSS123 FET is used to realize a level converter to 3.3V for each of the RX and TX signals.
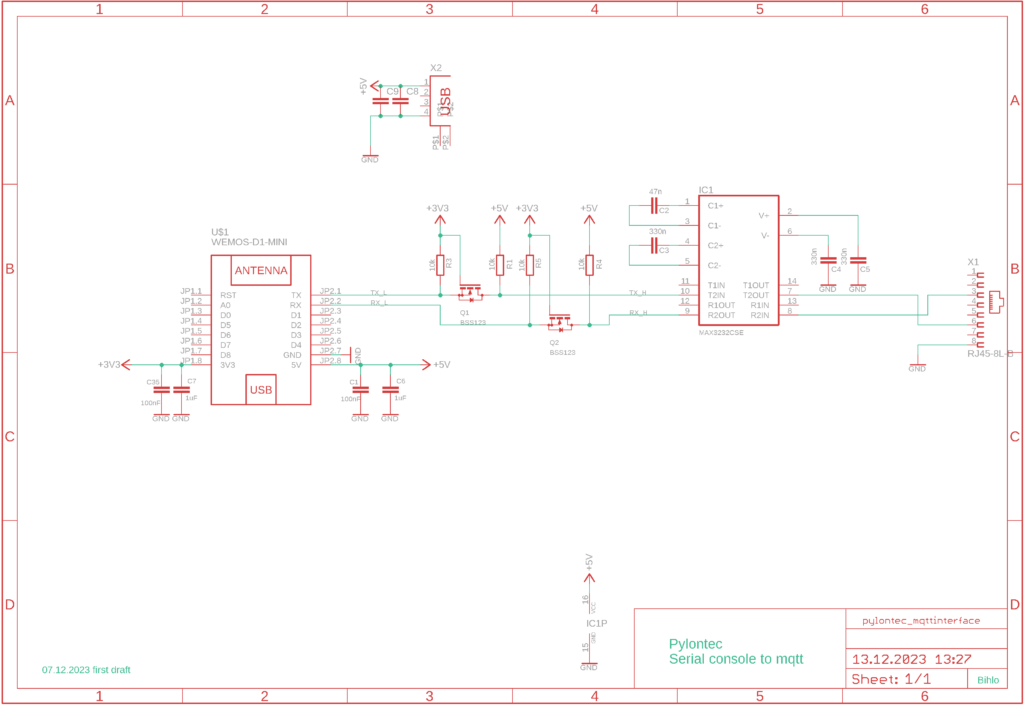
The ESP8266 processes this 3.3V TTL level in the form of the Wemos D1Mini or WemosD1Pro development board, which is plugged onto the circuit board. I then packed the entire construction into a small plastic housing, which can be conveniently connected to the Pylontec and a USB power source via the LAN and USB cables.
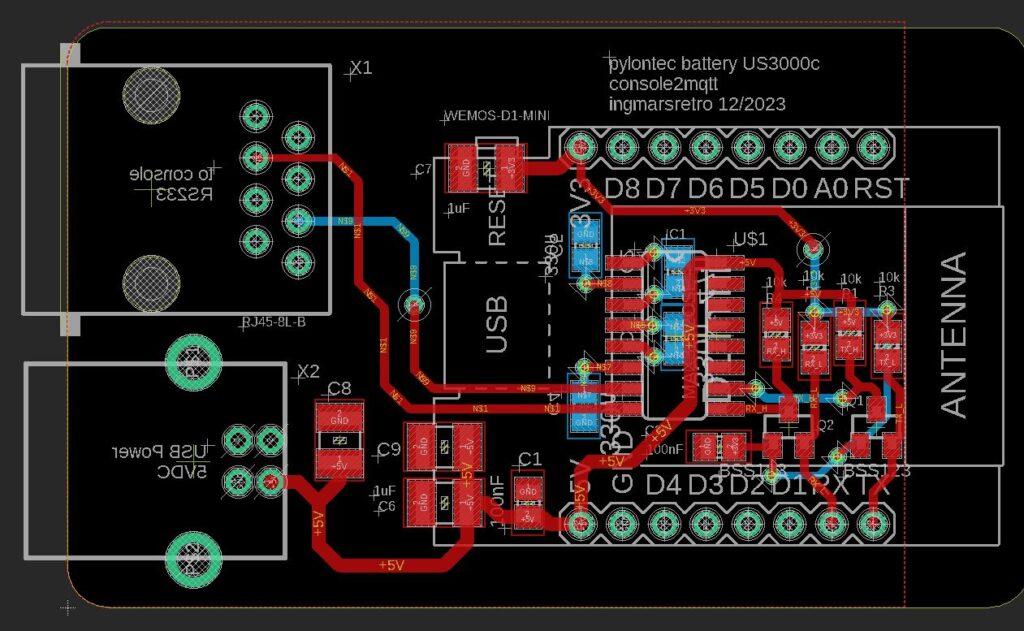
The layout design is shown in the picture above. The circuit board and the position of the components were checked again with the preview before production and then ordered from a trusted manufacturer.
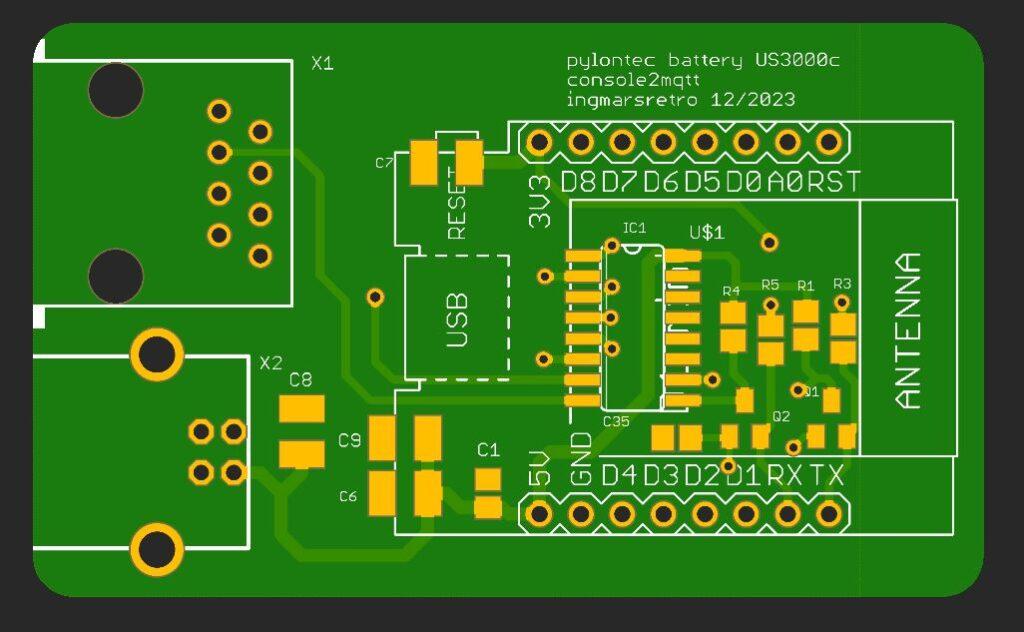
After barely two weeks of waiting, I had the empty circuit boards in my hands and was able to fit them with the components.
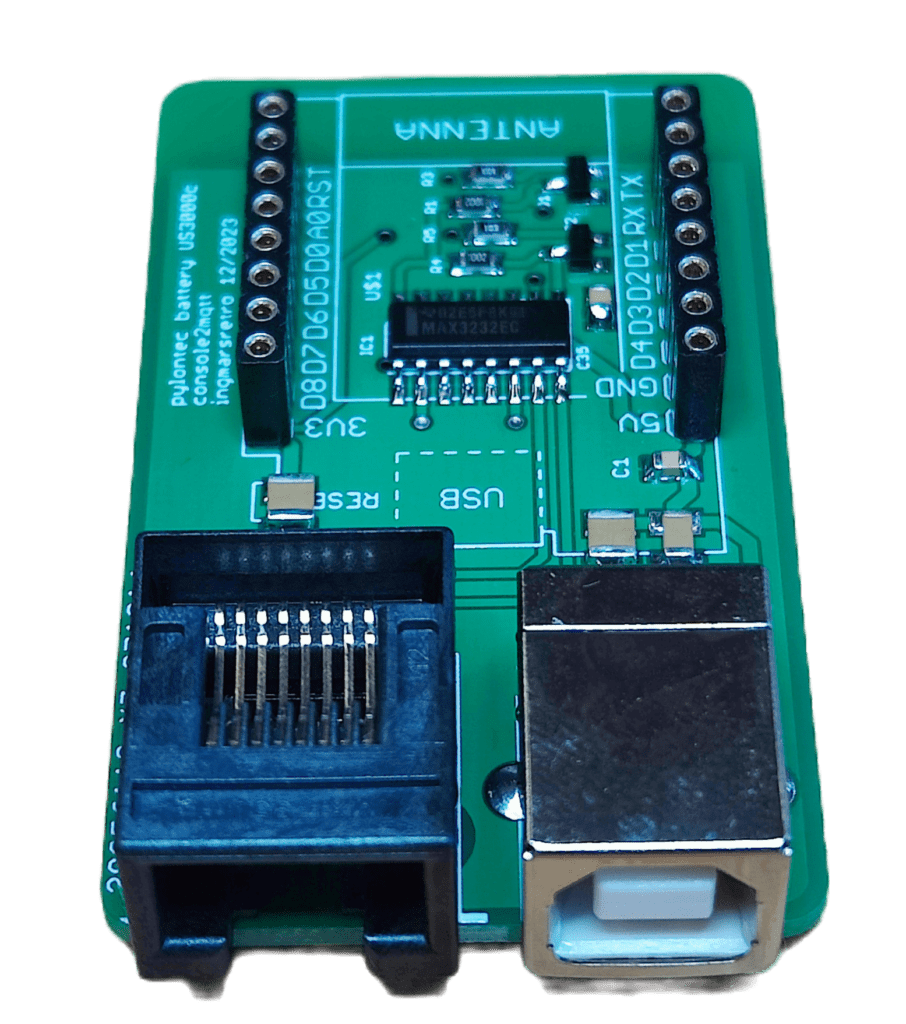
The picture above shows the fully assembled board. The only thing missing here is the Wemos board with the ESP.

In the end, I plugged in a WemosD1 Pro, as this offers the option of connecting an external WiFi antenna and thus getting a reasonable wireless range.
After flashing the software and commissioning, the Wemos web server can be accessed at the IP address specified in the code. Here you can also check whether the Pylontech battery is communicating with the Wemos. The result then looks like this.
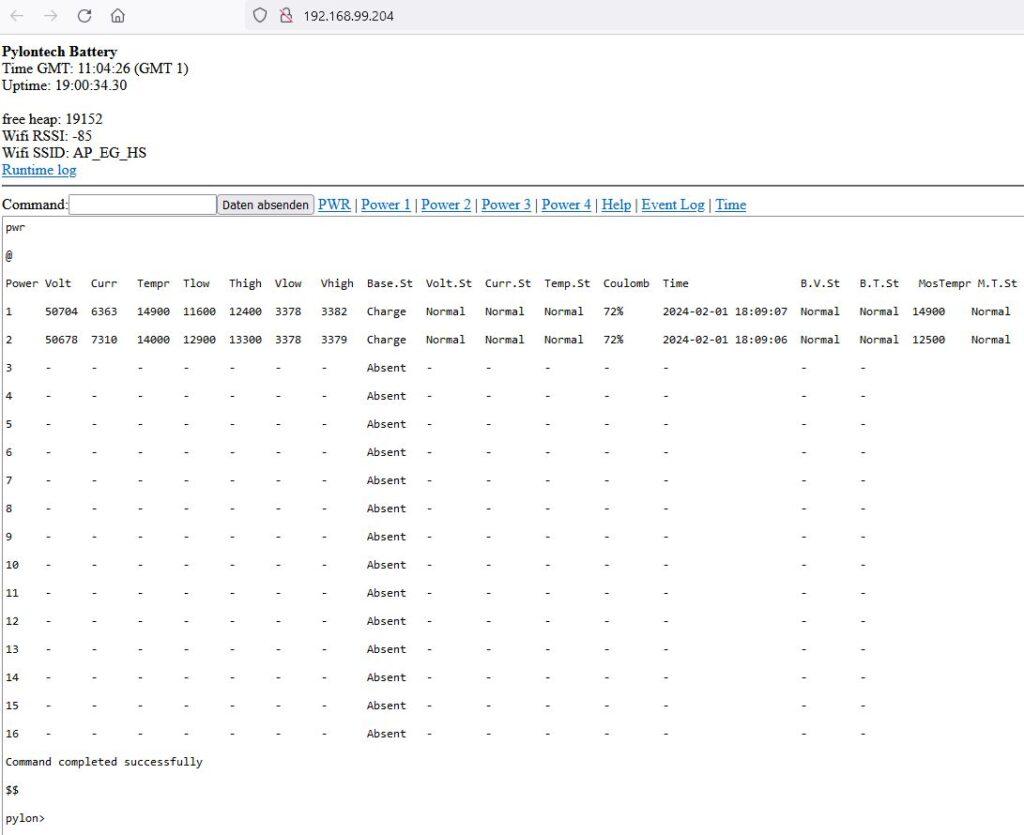
Here you can see that both battery modules are recognized correctly. The next step is to check whether messages are being sent via the MQTT protocol. The IP address of the MQTT broker must also be specified in the Wemo code. In my setup, I have set up the MQTT Explorer in the Home Assistant to be able to check the MQTT functions quickly and easily.
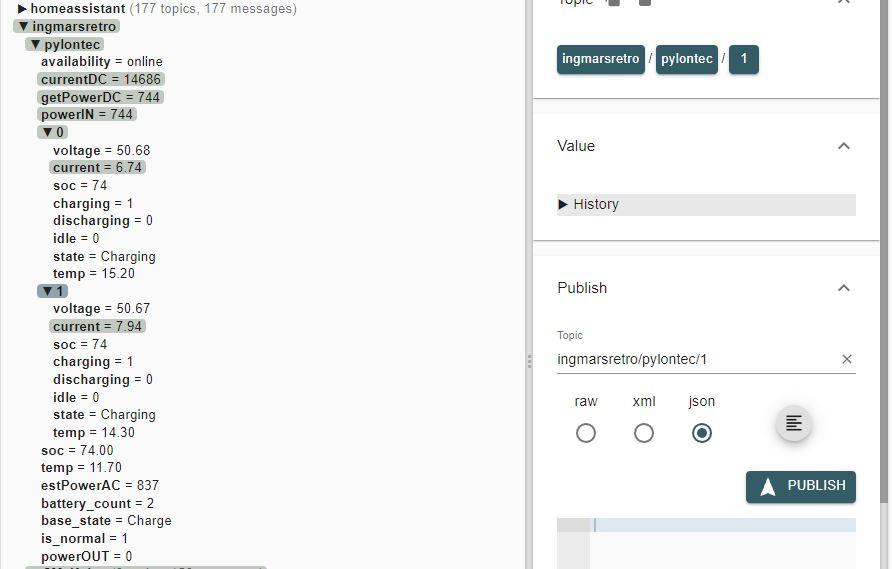
The image above shows that the data also arrives correctly via MQTT. Now it is only necessary to create a sensor yaml file in the home assistant to make the topics available as entities. I have added the following code to configuration.yaml for this purpose:
mqtt:
sensor:
#Pylontec Akku Serial Readout (ESP32 192.168.xxx.yyy)
- state_topic: "ingmarsretro/pylontec/ESP_WiFi_RSSI"
name: "Pylontec_RSSI"
unit_of_measurement: dB
- state_topic: "ingmarsretro/pylontec/availability"
name: "Pylontec_Status"
- state_topic: "ingmarsretro/pylontec/currentDC"
name: "DC-Strom"
unit_of_measurement: "mA"
- state_topic: "ingmarsretro/pylontec/getPowerDC"
name: "getPower DC"
unit_of_measurement: "W"
- state_topic: "ingmarsretro/pylontec/powerIN"
name: "Power IN"
unit_of_measurement: "W"
- state_topic: "ingmarsretro/pylontec/estPowerAC"
name: "Pylontec_estPowerAC"
unit_of_measurement: Watt
- state_topic: "ingmarsretro/pylontec/soc"
name: "Pylontec_SOC"
unit_of_measurement: "%"
- state_topic: "ingmarsretro/pylontec/temp"
name: "Pylontec_temperature"
unit_of_measurement: "°C"
- state_topic: "ingmarsretro/pylontec/battery_count"
name: "Pylontec_BatteryCount"
unit_of_measurement: "pcs"
- state_topic: "ingmarsretro/pylontec/base_state"
name: "Pylontec_BaseState"
- state_topic: "ingmarsretro/pylontec/is_normal"
name: "Pylontec_is_normal"
- state_topic: "ingmarsretro/pylontec/powerOUT"
name: "Pylontec_powerOUT"
unit_of_measurement: Watt
# Pylontech battery module 0
- state_topic: "ingmarsretro/pylontec/0/current"
name: "Pylontec_Battery0_current"
unit_of_measurement: "A"
- state_topic: "ingmarsretro/pylontec/0/voltage"
name: "Pylontec_Battery0_voltage"
unit_of_measurement: "V"
- state_topic: "ingmarsretro/pylontec/0/soc"
name: "Pylontec_Battery0_soc"
unit_of_measurement: "%"
- state_topic: "ingmarsretro/pylontec/0/charging"
name: "Pylontec_Battery0_charging"
- state_topic: "ingmarsretro/pylontec/0/discharging"
name: "Pylontec_Battery0_discharging"
- state_topic: "ingmarsretro/pylontec/0/idle"
name: "Pylontec_Battery0_idle"
- state_topic: "ingmarsretro/pylontec/0/state"
name: "Pylontec_Battery0_state"
- state_topic: "ingmarsretro/pylontec/0/temp"
name: "Pylontec_Battery0_temp"
unit_of_measurement: "°C"
# Pylontech battery module 1
- state_topic: "ingmarsretro/pylontec/1/current"
name: "Pylontec_Battery1_current"
unit_of_measurement: "A"
- state_topic: "ingmarsretro/pylontec/1/voltage"
name: "Pylontec_Battery1_voltage"
unit_of_measurement: "V"
- state_topic: "ingmarsretro/pylontec/1/soc"
name: "Pylontec_Battery1_soc"
unit_of_measurement: "%"
- state_topic: "ingmarsretro/pylontec/1/charging"
name: "Pylontec_Battery1_charging"
- state_topic: "ingmarsretro/pylontec/1/discharging"
name: "Pylontec_Battery1_discharging"
- state_topic: "ingmarsretro/pylontec/1/idle"
name: "Pylontec_Battery1_idle"
- state_topic: "ingmarsretro/pylontec/1/state"
name: "Pylontec_Battery1_state"
- state_topic: "ingmarsretro/pylontec/1/temp"
name: "Pylontec_Battery1_temp"
unit_of_measurement: "°C"
On the Homeassistant website, the visualization could then look like this, for example:
Last but not least, I am posting the customized code below. The libraries required for compilation and further information can be found in the GitHub links above.
#include <ESP8266WiFi.h>
#include <ESP8266mDNS.h>
#include <ArduinoOTA.h>
#include <ESP8266WebServer.h>
#include <circular_log.h>
#include <ArduinoJson.h>
#include <NTPClient.h>
#include <ESP8266TimerInterrupt.h>
//+++ START CONFIGURATION +++
//IMPORTANT: Specify your WIFI settings:
#define WIFI_SSID "wifiname"
#define WIFI_PASS deinpasswort1234"
#define WIFI_HOSTNAME "mppsolar-pylontec"
//Uncomment for static ip configuration
#define STATIC_IP
IPAddress local_IP(192, 168, xxx, yyy);
IPAddress subnet(255, 255, 255, 0);
IPAddress gateway(192, 168, xxx, zzz);
IPAddress primaryDNS(192, 168, xxx, zzz);
//Uncomment for authentication page
//#define AUTHENTICATION
//set http Authentication
const char* www_username = "admin";
const char* www_password = "password";
//IMPORTANT: Uncomment this line if you want to enable MQTT (and fill correct MQTT_ values below):
#define ENABLE_MQTT
// Set offset time in seconds to adjust for your timezone, for example:
// GMT +1 = 3600
// GMT +1 = 7200
// GMT +8 = 28800
// GMT -1 = -3600
// GMT 0 = 0
#define GMT 3600
//NOTE 1: if you want to change what is pushed via MQTT - edit function: pushBatteryDataToMqtt.
//NOTE 2: MQTT_TOPIC_ROOT is where battery will push MQTT topics. For example "soc" will be pushed to: "home/grid_battery/soc"
#define MQTT_SERVER "192.168.xx.broker"
#define MQTT_PORT 1883
#define MQTT_USER ""
#define MQTT_PASSWORD ""
#define MQTT_TOPIC_ROOT "ingmarsretro/pylontec/" //this is where mqtt data will be pushed
#define MQTT_PUSH_FREQ_SEC 2 //maximum mqtt update frequency in seconds
//+++ END CONFIGURATION +++
#ifdef ENABLE_MQTT
#include <PubSubClient.h>
WiFiClient espClient;
PubSubClient mqttClient(espClient);
#endif //ENABLE_MQTT
//text response
char g_szRecvBuff[7000];
const long utcOffsetInSeconds = GMT;
char daysOfTheWeek[7][12] = {"Sunday", "Monday", "Tuesday", "Wednesday", "Thursday", "Friday", "Saturday"};
// Define NTP Client to get time
WiFiUDP ntpUDP;
NTPClient timeClient(ntpUDP, "pool.ntp.org", utcOffsetInSeconds);
ESP8266WebServer server(80);
circular_log<7000> g_log;
bool ntpTimeReceived = false;
int g_baudRate = 0;
void Log(const char* msg)
{
g_log.Log(msg);
}
//Define Interrupt Timer to Calculate Power meter every second (kWh)
#define USING_TIM_DIV1 true // for shortest and most accurate timer
ESP8266Timer ITimer;
bool setInterval(unsigned long interval, timer_callback callback); // interval (in microseconds)
#define TIMER_INTERVAL_MS 1000
//Global Variables for the Power Meter - accessible from the calculating interrupt und from main
unsigned long powerIN = 0; //WS gone in to the BAttery
unsigned long powerOUT = 0; //WS gone out of the Battery
//Global Variables for the Power Meter - Überlauf
unsigned long powerINWh = 0; //WS gone in to the BAttery
unsigned long powerOUTWh = 0; //WS gone out of the Battery
void setup() {
memset(g_szRecvBuff, 0, sizeof(g_szRecvBuff)); //clean variable
pinMode(LED_BUILTIN, OUTPUT);
digitalWrite(LED_BUILTIN, HIGH);//high is off
// put your setup code here, to run once:
WiFi.mode(WIFI_STA);
WiFi.persistent(false); //our credentialss are hardcoded, so we don't need ESP saving those each boot (will save on flash wear)
WiFi.hostname(WIFI_HOSTNAME);
#ifdef STATIC_IP
WiFi.config(local_IP, gateway, subnet, primaryDNS);
#endif
WiFi.begin(WIFI_SSID, WIFI_PASS);
for(int ix=0; ix<10; ix++)
{
Log("Wait for WIFI Connection");
if(WiFi.status() == WL_CONNECTED)
{
break;
}
delay(1000);
}
ArduinoOTA.setHostname(WIFI_HOSTNAME);
ArduinoOTA.begin();
server.on("/", handleRoot);
server.on("/log", handleLog);
server.on("/req", handleReq);
server.on("/jsonOut", handleJsonOut);
server.on("/reboot", [](){
#ifdef AUTHENTICATION
if (!server.authenticate(www_username, www_password)) {
return server.requestAuthentication();
}
#endif
ESP.restart();
});
server.begin();
timeClient.begin();
#ifdef ENABLE_MQTT
mqttClient.setServer(MQTT_SERVER, MQTT_PORT);
#endif
Log("Boot event");
}
void handleLog()
{
#ifdef AUTHENTICATION
if (!server.authenticate(www_username, www_password)) {
return server.requestAuthentication();
}
#endif
server.send(200, "text/html", g_log.c_str());
}
void switchBaud(int newRate)
{
if(g_baudRate == newRate)
{
return;
}
if(g_baudRate != 0)
{
Serial.flush();
delay(20);
Serial.end();
delay(20);
}
char szMsg[50];
snprintf(szMsg, sizeof(szMsg)-1, "New baud: %d", newRate);
Log(szMsg);
Serial.begin(newRate);
g_baudRate = newRate;
delay(20);
}
void waitForSerial()
{
for(int ix=0; ix<150;ix++)
{
if(Serial.available()) break;
delay(10);
}
}
int readFromSerial()
{
memset(g_szRecvBuff, 0, sizeof(g_szRecvBuff));
int recvBuffLen = 0;
bool foundTerminator = true;
waitForSerial();
while(Serial.available())
{
char szResponse[256] = "";
const int readNow = Serial.readBytesUntil('>', szResponse, sizeof(szResponse)-1); //all commands terminate with "$$\r\n\rpylon>" (no new line at the end)
if(readNow > 0 &&
szResponse[0] != '\0')
{
if(readNow + recvBuffLen + 1 >= (int)(sizeof(g_szRecvBuff)))
{
Log("WARNING: Read too much data on the console!");
break;
}
strcat(g_szRecvBuff, szResponse);
recvBuffLen += readNow;
if(strstr(g_szRecvBuff, "$$\r\n\rpylon"))
{
strcat(g_szRecvBuff, ">"); //readBytesUntil will skip this, so re-add
foundTerminator = true;
break; //found end of the string
}
if(strstr(g_szRecvBuff, "Press [Enter] to be continued,other key to exit"))
{
//we need to send new line character so battery continues the output
Serial.write("\r");
}
waitForSerial();
}
}
if(recvBuffLen > 0 )
{
if(foundTerminator == false)
{
Log("Failed to find pylon> terminator");
}
}
return recvBuffLen;
}
bool readFromSerialAndSendResponse()
{
const int recvBuffLen = readFromSerial();
if(recvBuffLen > 0)
{
server.sendContent(g_szRecvBuff);
return true;
}
return false;
}
bool sendCommandAndReadSerialResponse(const char* pszCommand)
{
switchBaud(115200);
if(pszCommand[0] != '\0')
{
Serial.write(pszCommand);
}
Serial.write("\n");
const int recvBuffLen = readFromSerial();
if(recvBuffLen > 0)
{
return true;
}
//wake up console and try again:
wakeUpConsole();
if(pszCommand[0] != '\0')
{
Serial.write(pszCommand);
}
Serial.write("\n");
return readFromSerial() > 0;
}
void handleReq()
{
#ifdef AUTHENTICATION
if (!server.authenticate(www_username, www_password)) {
return server.requestAuthentication();
}
#endif
bool respOK;
if(server.hasArg("code") == false)
{
respOK = sendCommandAndReadSerialResponse("");
}
else
{
respOK = sendCommandAndReadSerialResponse(server.arg("code").c_str());
}
handleRoot();
}
void handleJsonOut()
{
#ifdef AUTHENTICATION
if (!server.authenticate(www_username, www_password)) {
return server.requestAuthentication();
}
#endif
if(sendCommandAndReadSerialResponse("pwr") == false)
{
server.send(500, "text/plain", "Failed to get response to 'pwr' command");
return;
}
parsePwrResponse(g_szRecvBuff);
prepareJsonOutput(g_szRecvBuff, sizeof(g_szRecvBuff));
server.send(200, "application/json", g_szRecvBuff);
}
void handleRoot() {
#ifdef AUTHENTICATION
if (!server.authenticate(www_username, www_password)) {
return server.requestAuthentication();
}
#endif
timeClient.update(); //get ntp datetime
unsigned long days = 0, hours = 0, minutes = 0;
unsigned long val = os_getCurrentTimeSec();
days = val / (3600*24);
val -= days * (3600*24);
hours = val / 3600;
val -= hours * 3600;
minutes = val / 60;
val -= minutes*60;
time_t epochTime = timeClient.getEpochTime();
String formattedTime = timeClient.getFormattedTime();
//Get a time structure
struct tm *ptm = gmtime ((time_t *)&epochTime);
int currentMonth = ptm->tm_mon+1;
static char szTmp[9500] = "";
long timezone= GMT / 3600;
snprintf(szTmp, sizeof(szTmp)-1, "<html><b>Pylontech Battery</b><br>Time GMT: %s (%s %d)<br>Uptime: %02d:%02d:%02d.%02d<br><br>free heap: %u<br>Wifi RSSI: %d<BR>Wifi SSID: %s",
formattedTime, "GMT ", timezone,
(int)days, (int)hours, (int)minutes, (int)val,
ESP.getFreeHeap(), WiFi.RSSI(), WiFi.SSID().c_str());
strncat(szTmp, "<BR><a href='/log'>Runtime log</a><HR>", sizeof(szTmp)-1);
strncat(szTmp, "<form action='/req' method='get'>Command:<input type='text' name='code'/><input type='submit'> <a href='/req?code=pwr'>PWR</a> | <a href='/req?code=pwr%201'>Power 1</a> | <a href='/req?code=pwr%202'>Power 2</a> | <a href='/req?code=pwr%203'>Power 3</a> | <a href='/req?code=pwr%204'>Power 4</a> | <a href='/req?code=help'>Help</a> | <a href='/req?code=log'>Event Log</a> | <a href='/req?code=time'>Time</a><br>", sizeof(szTmp)-1);
//strncat(szTmp, "<form action='/req' method='get'>Command:<input type='text' name='code'/><input type='submit'><a href='/req?code=pwr'>Power</a> | <a href='/req?code=help'>Help</a> | <a href='/req?code=log'>Event Log</a> | <a href='/req?code=time'>Time</a><br>", sizeof(szTmp)-1);
strncat(szTmp, "<textarea rows='80' cols='180'>", sizeof(szTmp)-1);
//strncat(szTmp, "<textarea rows='45' cols='180'>", sizeof(szTmp)-1);
strncat(szTmp, g_szRecvBuff, sizeof(szTmp)-1);
strncat(szTmp, "</textarea></form>", sizeof(szTmp)-1);
strncat(szTmp, "</html>", sizeof(szTmp)-1);
//send page
server.send(200, "text/html", szTmp);
}
unsigned long os_getCurrentTimeSec()
{
static unsigned int wrapCnt = 0;
static unsigned long lastVal = 0;
unsigned long currentVal = millis();
if(currentVal < lastVal)
{
wrapCnt++;
}
lastVal = currentVal;
unsigned long seconds = currentVal/1000;
//millis will wrap each 50 days, as we are interested only in seconds, let's keep the wrap counter
return (wrapCnt*4294967) + seconds;
}
void wakeUpConsole()
{
switchBaud(1200);
//byte wakeUpBuff[] = {0x7E, 0x32, 0x30, 0x30, 0x31, 0x34, 0x36, 0x38, 0x32, 0x43, 0x30, 0x30, 0x34, 0x38, 0x35, 0x32, 0x30, 0x46, 0x43, 0x43, 0x33, 0x0D};
//Serial.write(wakeUpBuff, sizeof(wakeUpBuff));
Serial.write("~20014682C0048520FCC3\r");
delay(1000);
byte newLineBuff[] = {0x0E, 0x0A};
switchBaud(115200);
for(int ix=0; ix<10; ix++)
{
Serial.write(newLineBuff, sizeof(newLineBuff));
delay(1000);
if(Serial.available())
{
while(Serial.available())
{
Serial.read();
}
break;
}
}
}
#define MAX_PYLON_BATTERIES 8
struct pylonBattery
{
bool isPresent;
long soc; //Coulomb in %
long voltage; //in mW
long current; //in mA, negative value is discharge
long tempr; //temp of case or BMS?
long cellTempLow;
long cellTempHigh;
long cellVoltLow;
long cellVoltHigh;
char baseState[9]; //Charge | Dischg | Idle
char voltageState[9]; //Normal
char currentState[9]; //Normal
char tempState[9]; //Normal
char time[20]; //2019-06-08 04:00:29
char b_v_st[9]; //Normal (battery voltage?)
char b_t_st[9]; //Normal (battery temperature?)
bool isCharging() const { return strcmp(baseState, "Charge") == 0; }
bool isDischarging() const { return strcmp(baseState, "Dischg") == 0; }
bool isIdle() const { return strcmp(baseState, "Idle") == 0; }
bool isBalancing() const { return strcmp(baseState, "Balance") == 0; }
bool isNormal() const
{
if(isCharging() == false &&
isDischarging() == false &&
isIdle() == false &&
isBalancing() == false)
{
return false; //base state looks wrong!
}
return strcmp(voltageState, "Normal") == 0 &&
strcmp(currentState, "Normal") == 0 &&
strcmp(tempState, "Normal") == 0 &&
strcmp(b_v_st, "Normal") == 0 &&
strcmp(b_t_st, "Normal") == 0 ;
}
};
struct batteryStack
{
int batteryCount;
int soc; //in %, if charging: average SOC, otherwise: lowest SOC
int temp; //in mC, if highest temp is > 15C, this will show the highest temp, otherwise the lowest
long currentDC; //mAh current going in or out of the battery
long avgVoltage; //in mV
char baseState[9]; //Charge | Dischg | Idle | Balance | Alarm!
pylonBattery batts[MAX_PYLON_BATTERIES];
bool isNormal() const
{
for(int ix=0; ix<MAX_PYLON_BATTERIES; ix++)
{
if(batts[ix].isPresent &&
batts[ix].isNormal() == false)
{
return false;
}
}
return true;
}
//in Wh
long getPowerDC() const
{
return (long)(((double)currentDC/1000.0)*((double)avgVoltage/1000.0));
}
// power in Wh in charge
float powerIN() const
{
if (currentDC > 0) {
return (float)(((double)currentDC/1000.0)*((double)avgVoltage/1000.0));
} else {
return (float)(0);
}
}
// power in Wh in discharge
float powerOUT() const
{
if (currentDC < 0) {
return (float)(((double)currentDC/1000.0)*((double)avgVoltage/1000.0)*-1);
} else {
return (float)(0);
}
}
//Wh estimated current on AC side (taking into account Sofar ME3000SP losses)
long getEstPowerAc() const
{
double powerDC = (double)getPowerDC();
if(powerDC == 0)
{
return 0;
}
else if(powerDC < 0)
{
//we are discharging, on AC side we will see less power due to losses
if(powerDC < -1000)
{
return (long)(powerDC*0.94);
}
else if(powerDC < -600)
{
return (long)(powerDC*0.90);
}
else
{
return (long)(powerDC*0.87);
}
}
else
{
//we are charging, on AC side we will have more power due to losses
if(powerDC > 1000)
{
return (long)(powerDC*1.06);
}
else if(powerDC > 600)
{
return (long)(powerDC*1.1);
}
else
{
return (long)(powerDC*1.13);
}
}
}
};
batteryStack g_stack;
long extractInt(const char* pStr, int pos)
{
return atol(pStr+pos);
}
void extractStr(const char* pStr, int pos, char* strOut, int strOutSize)
{
strOut[strOutSize-1] = '\0';
strncpy(strOut, pStr+pos, strOutSize-1);
strOutSize--;
//trim right
while(strOutSize > 0)
{
if(isspace(strOut[strOutSize-1]))
{
strOut[strOutSize-1] = '\0';
}
else
{
break;
}
strOutSize--;
}
}
/* Output has mixed \r and \r\n
pwr
@
Power Volt Curr Tempr Tlow Thigh Vlow Vhigh Base.St Volt.St Curr.St Temp.St Coulomb Time B.V.St B.T.St
1 49735 -1440 22000 19000 19000 3315 3317 Dischg Normal Normal Normal 93% 2019-06-08 04:00:30 Normal Normal
....
8 - - - - - - - Absent - - - - - - -
Command completed successfully
$$
pylon
*/
bool parsePwrResponse(const char* pStr)
{
if(strstr(pStr, "Command completed successfully") == NULL)
{
return false;
}
int chargeCnt = 0;
int dischargeCnt = 0;
int idleCnt = 0;
int alarmCnt = 0;
int socAvg = 0;
int socLow = 0;
int tempHigh = 0;
int tempLow = 0;
memset(&g_stack, 0, sizeof(g_stack));
for(int ix=0; ix<MAX_PYLON_BATTERIES; ix++)
{
char szToFind[32] = "";
snprintf(szToFind, sizeof(szToFind)-1, "\r\r\n%d ", ix+1);
const char* pLineStart = strstr(pStr, szToFind);
if(pLineStart == NULL)
{
return false;
}
pLineStart += 3; //move past \r\r\n
extractStr(pLineStart, 55, g_stack.batts[ix].baseState, sizeof(g_stack.batts[ix].baseState));
if(strcmp(g_stack.batts[ix].baseState, "Absent") == 0)
{
g_stack.batts[ix].isPresent = false;
}
else
{
g_stack.batts[ix].isPresent = true;
extractStr(pLineStart, 64, g_stack.batts[ix].voltageState, sizeof(g_stack.batts[ix].voltageState));
extractStr(pLineStart, 73, g_stack.batts[ix].currentState, sizeof(g_stack.batts[ix].currentState));
extractStr(pLineStart, 82, g_stack.batts[ix].tempState, sizeof(g_stack.batts[ix].tempState));
extractStr(pLineStart, 100, g_stack.batts[ix].time, sizeof(g_stack.batts[ix].time));
extractStr(pLineStart, 121, g_stack.batts[ix].b_v_st, sizeof(g_stack.batts[ix].b_v_st));
extractStr(pLineStart, 130, g_stack.batts[ix].b_t_st, sizeof(g_stack.batts[ix].b_t_st));
g_stack.batts[ix].voltage = extractInt(pLineStart, 6);
g_stack.batts[ix].current = extractInt(pLineStart, 13);
g_stack.batts[ix].tempr = extractInt(pLineStart, 20);
g_stack.batts[ix].cellTempLow = extractInt(pLineStart, 27);
g_stack.batts[ix].cellTempHigh = extractInt(pLineStart, 34);
g_stack.batts[ix].cellVoltLow = extractInt(pLineStart, 41);
g_stack.batts[ix].cellVoltHigh = extractInt(pLineStart, 48);
g_stack.batts[ix].soc = extractInt(pLineStart, 91);
//////////////////////////////// Post-process ////////////////////////
g_stack.batteryCount++;
g_stack.currentDC += g_stack.batts[ix].current;
g_stack.avgVoltage += g_stack.batts[ix].voltage;
socAvg += g_stack.batts[ix].soc;
if(g_stack.batts[ix].isNormal() == false){ alarmCnt++; }
else if(g_stack.batts[ix].isCharging()){chargeCnt++;}
else if(g_stack.batts[ix].isDischarging()){dischargeCnt++;}
else if(g_stack.batts[ix].isIdle()){idleCnt++;}
else{ alarmCnt++; } //should not really happen!
if(g_stack.batteryCount == 1)
{
socLow = g_stack.batts[ix].soc;
tempLow = g_stack.batts[ix].cellTempLow;
tempHigh = g_stack.batts[ix].cellTempHigh;
}
else
{
if(socLow > g_stack.batts[ix].soc){socLow = g_stack.batts[ix].soc;}
if(tempHigh < g_stack.batts[ix].cellTempHigh){tempHigh = g_stack.batts[ix].cellTempHigh;}
if(tempLow > g_stack.batts[ix].cellTempLow){tempLow = g_stack.batts[ix].cellTempLow;}
}
}
}
//now update stack state:
g_stack.avgVoltage /= g_stack.batteryCount;
g_stack.soc = socLow;
if(tempHigh > 15000) //15C
{
g_stack.temp = tempHigh; //in the summer we highlight the warmest cell
}
else
{
g_stack.temp = tempLow; //in the winter we focus on coldest cell
}
if(alarmCnt > 0)
{
strcpy(g_stack.baseState, "Alarm!");
}
else if(chargeCnt == g_stack.batteryCount)
{
strcpy(g_stack.baseState, "Charge");
g_stack.soc = (int)(socAvg / g_stack.batteryCount);
}
else if(dischargeCnt == g_stack.batteryCount)
{
strcpy(g_stack.baseState, "Dischg");
}
else if(idleCnt == g_stack.batteryCount)
{
strcpy(g_stack.baseState, "Idle");
}
else
{
strcpy(g_stack.baseState, "Balance");
}
return true;
}
void prepareJsonOutput(char* pBuff, int buffSize)
{
memset(pBuff, 0, buffSize);
snprintf(pBuff, buffSize-1, "{\"soc\": %d, \"temp\": %d, \"currentDC\": %ld, \"avgVoltage\": %ld, \"baseState\": \"%s\", \"batteryCount\": %d, \"powerDC\": %ld, \"estPowerAC\": %ld, \"isNormal\": %s}", g_stack.soc,
g_stack.temp,
g_stack.currentDC,
g_stack.avgVoltage,
g_stack.baseState,
g_stack.batteryCount,
g_stack.getPowerDC(),
g_stack.getEstPowerAc(),
g_stack.isNormal() ? "true" : "false");
}
void loop() {
#ifdef ENABLE_MQTT
mqttLoop();
#endif
ArduinoOTA.handle();
server.handleClient();
//if there are bytes availbe on serial here - it's unexpected
//when we send a command to battery, we read whole response
//if we get anything here anyways - we will log it
int bytesAv = Serial.available();
if(bytesAv > 0)
{
if(bytesAv > 63)
{
bytesAv = 63;
}
char buff[64+4] = "RCV:";
if(Serial.readBytes(buff+4, bytesAv) > 0)
{
digitalWrite(LED_BUILTIN, LOW);
delay(5);
digitalWrite(LED_BUILTIN, HIGH);//high is off
Log(buff);
}
}
}
#ifdef ENABLE_MQTT
#define ABS_DIFF(a, b) (a > b ? a-b : b-a)
void mqtt_publish_f(const char* topic, float newValue, float oldValue, float minDiff, bool force)
{
char szTmp[16] = "";
snprintf(szTmp, 15, "%.2f", newValue);
if(force || ABS_DIFF(newValue, oldValue) > minDiff)
{
mqttClient.publish(topic, szTmp, false);
}
}
void mqtt_publish_i(const char* topic, int newValue, int oldValue, int minDiff, bool force)
{
char szTmp[16] = "";
snprintf(szTmp, 15, "%d", newValue);
if(force || ABS_DIFF(newValue, oldValue) > minDiff)
{
mqttClient.publish(topic, szTmp, false);
}
}
void mqtt_publish_s(const char* topic, const char* newValue, const char* oldValue, bool force)
{
if(force || strcmp(newValue, oldValue) != 0)
{
mqttClient.publish(topic, newValue, false);
}
}
void pushBatteryDataToMqtt(const batteryStack& lastSentData, bool forceUpdate /* if true - we will send all data regardless if it's the same */)
{
mqtt_publish_f(MQTT_TOPIC_ROOT "soc", g_stack.soc, lastSentData.soc, 0, forceUpdate);
mqtt_publish_f(MQTT_TOPIC_ROOT "temp", (float)g_stack.temp/1000.0, (float)lastSentData.temp/1000.0, 0.1, forceUpdate);
mqtt_publish_i(MQTT_TOPIC_ROOT "currentDC", g_stack.currentDC, lastSentData.currentDC, 1, forceUpdate);
mqtt_publish_i(MQTT_TOPIC_ROOT "estPowerAC", g_stack.getEstPowerAc(), lastSentData.getEstPowerAc(), 10, forceUpdate);
mqtt_publish_i(MQTT_TOPIC_ROOT "battery_count",g_stack.batteryCount, lastSentData.batteryCount, 0, forceUpdate);
mqtt_publish_s(MQTT_TOPIC_ROOT "base_state", g_stack.baseState, lastSentData.baseState , forceUpdate);
mqtt_publish_i(MQTT_TOPIC_ROOT "is_normal", g_stack.isNormal() ? 1:0, lastSentData.isNormal() ? 1:0, 0, forceUpdate);
mqtt_publish_i(MQTT_TOPIC_ROOT "getPowerDC", g_stack.getPowerDC(), lastSentData.getPowerDC(), 1, forceUpdate);
mqtt_publish_i(MQTT_TOPIC_ROOT "powerIN", g_stack.powerIN(), lastSentData.powerIN(), 1, forceUpdate);
mqtt_publish_i(MQTT_TOPIC_ROOT "powerOUT", g_stack.powerOUT(), lastSentData.powerOUT(), 1, forceUpdate);
// publishing details
for (int ix = 0; ix < g_stack.batteryCount; ix++) {
char ixBuff[50];
String ixBattStr = MQTT_TOPIC_ROOT + String(ix) + "/voltage";
ixBattStr.toCharArray(ixBuff, 50);
mqtt_publish_f(ixBuff, g_stack.batts[ix].voltage / 1000.0, lastSentData.batts[ix].voltage / 1000.0, 0, forceUpdate);
ixBattStr = MQTT_TOPIC_ROOT + String(ix) + "/current";
ixBattStr.toCharArray(ixBuff, 50);
mqtt_publish_f(ixBuff, g_stack.batts[ix].current / 1000.0, lastSentData.batts[ix].current / 1000.0, 0, forceUpdate);
ixBattStr = MQTT_TOPIC_ROOT + String(ix) + "/soc";
ixBattStr.toCharArray(ixBuff, 50);
mqtt_publish_i(ixBuff, g_stack.batts[ix].soc, lastSentData.batts[ix].soc, 0, forceUpdate);
ixBattStr = MQTT_TOPIC_ROOT + String(ix) + "/charging";
ixBattStr.toCharArray(ixBuff, 50);
mqtt_publish_i(ixBuff, g_stack.batts[ix].isCharging()?1:0, lastSentData.batts[ix].isCharging()?1:0, 0, forceUpdate);
ixBattStr = MQTT_TOPIC_ROOT + String(ix) + "/discharging";
ixBattStr.toCharArray(ixBuff, 50);
mqtt_publish_i(ixBuff, g_stack.batts[ix].isDischarging()?1:0, lastSentData.batts[ix].isDischarging()?1:0, 0, forceUpdate);
ixBattStr = MQTT_TOPIC_ROOT + String(ix) + "/idle";
ixBattStr.toCharArray(ixBuff, 50);
mqtt_publish_i(ixBuff, g_stack.batts[ix].isIdle()?1:0, lastSentData.batts[ix].isIdle()?1:0, 0, forceUpdate);
ixBattStr = MQTT_TOPIC_ROOT + String(ix) + "/state";
ixBattStr.toCharArray(ixBuff, 50);
mqtt_publish_s(ixBuff, g_stack.batts[ix].isIdle()?"Idle":g_stack.batts[ix].isCharging()?"Charging":g_stack.batts[ix].isDischarging()?"Discharging":"", lastSentData.batts[ix].isIdle()?"Idle":lastSentData.batts[ix].isCharging()?"Charging":lastSentData.batts[ix].isDischarging()?"Discharging":"", forceUpdate);
ixBattStr = MQTT_TOPIC_ROOT + String(ix) + "/temp";
ixBattStr.toCharArray(ixBuff, 50);
mqtt_publish_f(ixBuff, (float)g_stack.batts[ix].tempr/1000.0, (float)lastSentData.batts[ix].tempr/1000.0, 0.1, forceUpdate);
}
}
void mqttLoop()
{
//if we have problems with connecting to mqtt server, we will attempt to re-estabish connection each 1minute (not more than that)
static unsigned long g_lastConnectionAttempt = 0;
//first: let's make sure we are connected to mqtt
const char* topicLastWill = MQTT_TOPIC_ROOT "availability";
if (!mqttClient.connected() && (g_lastConnectionAttempt == 0 || os_getCurrentTimeSec() - g_lastConnectionAttempt > 60)) {
if(mqttClient.connect(WIFI_HOSTNAME, MQTT_USER, MQTT_PASSWORD, topicLastWill, 1, true, "offline"))
{
Log("Connected to MQTT server: " MQTT_SERVER);
mqttClient.publish(topicLastWill, "online", true);
}
else
{
Log("Failed to connect to MQTT server.");
}
g_lastConnectionAttempt = os_getCurrentTimeSec();
}
//next: read data from battery and send via MQTT (but only once per MQTT_PUSH_FREQ_SEC seconds)
static unsigned long g_lastDataSent = 0;
if(mqttClient.connected() &&
os_getCurrentTimeSec() - g_lastDataSent > MQTT_PUSH_FREQ_SEC &&
sendCommandAndReadSerialResponse("pwr") == true)
{
static batteryStack lastSentData; //this is the last state we sent to MQTT, used to prevent sending the same data over and over again
static unsigned int callCnt = 0;
parsePwrResponse(g_szRecvBuff);
bool forceUpdate = (callCnt % 20 == 0); //push all the data every 20th call
pushBatteryDataToMqtt(lastSentData, forceUpdate);
callCnt++;
g_lastDataSent = os_getCurrentTimeSec();
memcpy(&lastSentData, &g_stack, sizeof(batteryStack));
}
mqttClient.loop();
}
#endif //ENABLE_MQTT